Research interest in our lab can be broadly defined in the field of Applied Optics and Laser Sciences. Current focus areas are as follows:
Supersymmetry Photonics and Lasers
Non-Hermitian Optics and Sensors
Photonic Crystals and Metamaterials for Sensing, Communications, and Imaging
- https://doi.org/10.1002/adma.202401344
- https://doi.org/10.1103/PhysRevApplied.1.044003
- https://doi.org/10.1038/srep14373,
- https://doi.org/10.1016/j.ijleo.2011.03.013
Optical Sensors
We employ emerging photonic structures such as Metamaterials and Photonic Crystals within active and passive platforms and we leverage concepts from fields such as quantum condensed matter area, to observe new optical phenomena and to enhance performance characteristics of optical devices. For one example, concepts from Supersymmetry have been successfully utilized to address a long-standing challenge in laser science, in-phase locking of semiconductor laser arrays for brightness enhancement. For another example, notions from non-Hermitian Quantum systems have been deployed to boost sensitivity of ring laser gyroscopes.
The research in our lab is both theoretical and experimental and is interdisciplinary by its nature. We use theoretical and mathematical models as well as software tools to design next generations of optical devices. Meanwhile, intelligent techniques such as AI, Particle Swamp, and Genetic Algorithm methods may also be applied for optimization. The devices are then made using micro/nano fabrication techniques. We also design and build our test setups to validate the performance of the devices. Being in touch with industrial partners for collaborations and professional student trainings, is also one of the priorities. Upon graduation, students in my group are expected to develop knowledge and skills not only in Optics but also in micro/nano fabrications, and device characterization.
Please see below for more details regarding some of the research topics.
Supersymmetry Photonics and Lasers
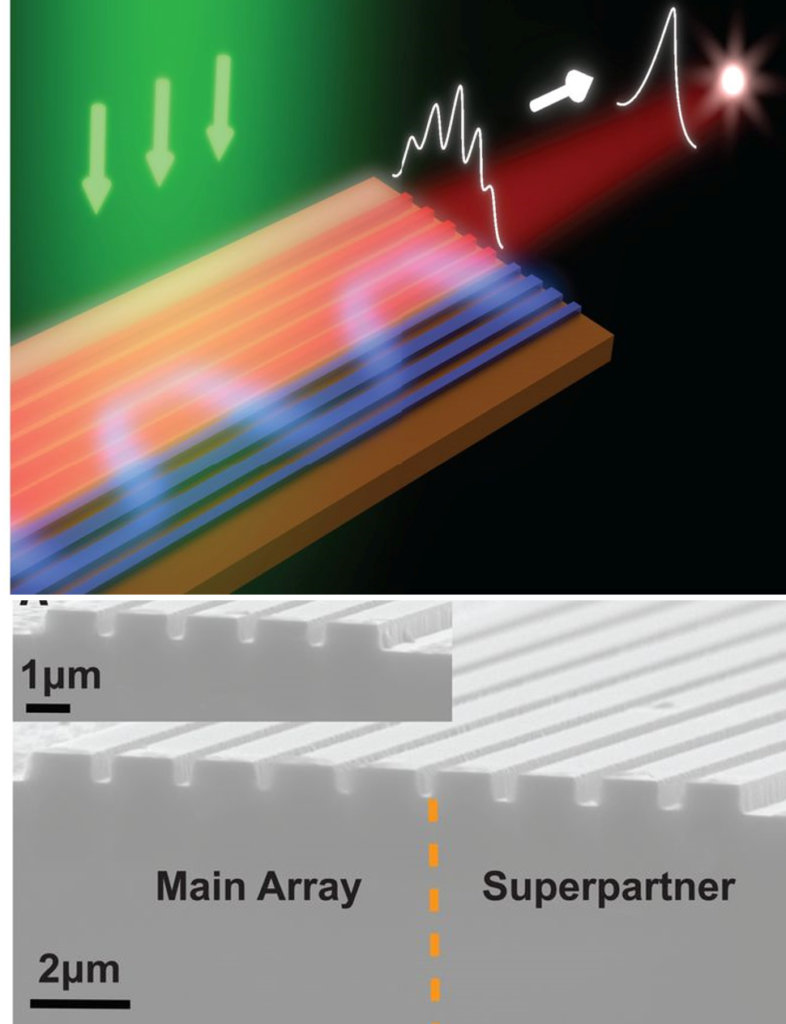
Supersymmetry (SUSY) is a theory that was first proposed in high energy physics as an extension of the standard model in order to resolve several unanswered questions in this area. The theoretical framework of SUSY has been adopted in Optics. SUSY has found implications in Transformation Optics, communication systems, as well as in Laser Science. For example, using this technique one can create optical structures with entirely different index profiles but with similar scattering behaviors. For another example, one can use this approach to mimic scattering characteristics of high contrast structures using solely low contrast optical materials. We have also experimentally exhibited a SUSY semiconductor laser arrays that operate exclusively in their in-phase mode with enhanced brightness. This was a long-standing question in laser science that was addressed using SUSY technique. (Demonstrated when Hokmabadi was with UCF and USC in Khajavikhan’s group)
Courtesy of Profs. M. Kahjavikhan, D. Christodoulides and Dr. M. Kanskar
Non-Hermitian Optics
When dealing with physical systems, we often assume that the Hamiltonian defining their response is Hermitian with real eigen values. It was believed for a long time that non-Hermitian open settings can only support complex eigen energies. Recently however, it has been found that there is a large subset of non-Hermitian arrangements that hold real eigen spectrum, provided that their Hamiltonian possess simultaneous symmetry in space and time. This particular symmetry is known as parity-time (PT) symmetry which can be spontaneously broken. Interestingly, the break in this symmetry is associated with a singular point in their parameter space, called Exceptional Point (EP). EP-based systems are a host of exciting properties, one of which is an enhanced sensitivity of their response. We adopted notions from non-Hermitian Optics, and judicially modified the cavity of a standard ring laser gyroscope to create such a singularity. Doing so, we were able to enhance the rotation sensitivity of RLGs by an order of magnitude in the presence o f EP. RLGs are among the most sensitive devices that are used for rotation sensing. (Demonstrated when Hokmabadi was with UCF and USC in Khajavikhan’s group)
Courtesy of Profs. M. Kahjavikhan and D. Christodoulides
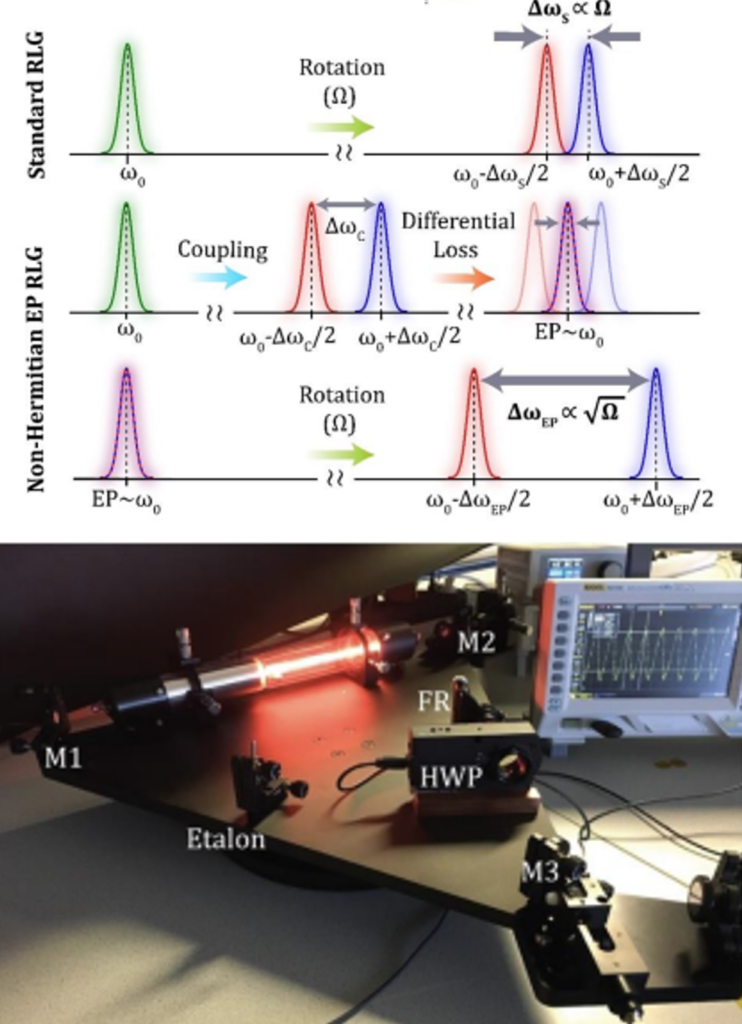
Metamaterials and Photonic Crystals
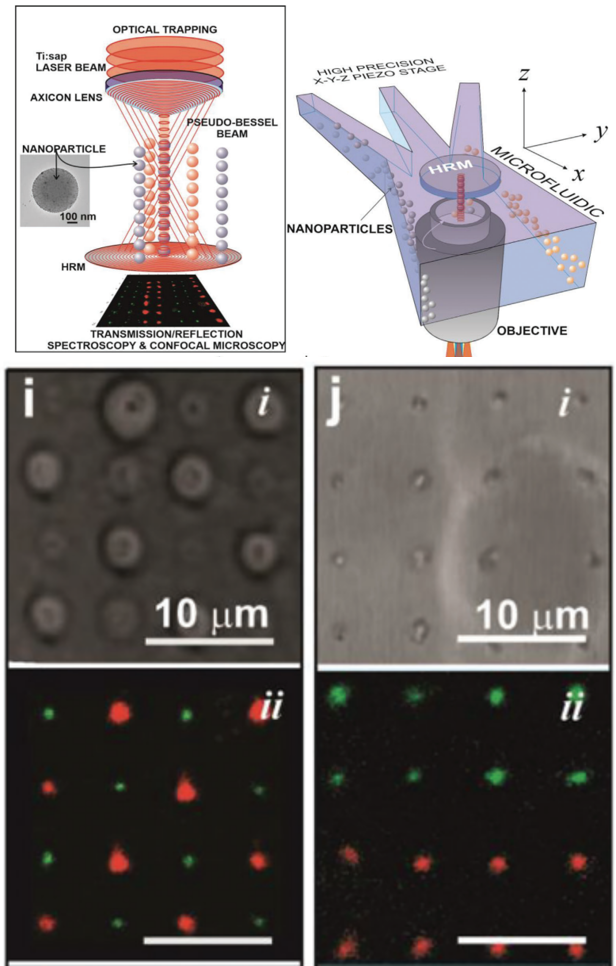
Often times, scarcity of natural materials with effective optical responses hinders technological advances. This raises the question of whether one can build a structure using available natural substances, that can demonstrate unseen optical characteristics. Metamaterials and Photonic Crystals are among those configurations. Not only they can exhibit unusual optical properties not found in nature, but they can also mimic certain well-known quantum condensed matter phenomena which would be otherwise hard to implement. Invisibility cloak, superlensing, nano lasers, flat lenses, and high power Photonic Crystal Surface Emitting Lasers (PCSEL) are some of the wide range applications of these exotic materials. While being in S. M. Kim’s Lab, we have contributed to building several Metamaterials structures for Terahertz frequency band, such as Stereometamaterial Absorber, Liquid Crystal Tunable Absorber and Slow-Light Metamaterial employing standard lithography techniques. Alternatively, our group has contributed to constructing all dielectric Metamaterials as well as Photonic Crystals utilizing the power of optical tweezers. In this particular effort, which was done in Timp’s Lab in University of Notre Dame, optical tweezers were used to form homogeneous and heterogeneous assembly of Metamaterials and Photonic Crystals. (Demonstrated when Hokmabadi was with University of Notre Dame in Timp’s group)
Courtesy of Profs. G. Timp, S. M. Kim , and P. Kung