Multiscale Modeling of Nanomaterials
Nanomaterials are defined as materials whose main constituents have a dimension less than 100 nm. Example of nanomaterials include quantum dots, nanotubes and nanoparticle reinforced nanocomposites. Novel mechanical and electronic properties of nanomaterials have been identified as the key to the production of next generation of devices with revolutionary functionalities.
Computer simulations can play a prominent role in understanding the connection between the atomic structure and the macroscopic properties of nanomaterials. We adopt a multiscale approach to develop robust computational techniques to predict the mechanical properties and behavior of systems which contain nanomaterials. The main challenge is to bridge multiple time and length scales in order to be able to pass from atoms to microstructures and finally to macroscale. Thorough this research we develop MD-Continuum or quantum-continuum coupling techniques to simulate material behavior under static or dynamic loading.
Computational Simulations of Dynamic Failure of Materials
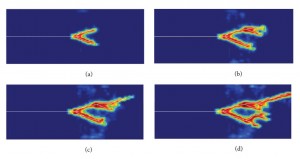
Using XFEM to model dynamic crack branching and growth
Dynamic failure mechanics is the study of the failure of materials under high strain rates. Examples of dynamic failure in the real world are vehicle crash, high speed machining and objects impact. Dynamic failure divides into two major categories: dynamic fracture and adiabatic shear bands (ADBs). We utilize micro-macro continuum and atomistic-continuum multiscale methods to model failure of material under intense dynamic loads. In this research extended finite element method (XFEM) is used to model material fracture at the macroscale, and micro/atomisitc level simulations are used to obtain the cohesive forces acting on the shear bands/cracks. The goal is to gain insights about the micromechanisms of dynamic failure of ductile materials and to design materials with superior dynamic properties.
Atomistic Modeling of the Mechanical Properties of Two-Dimensional Materials
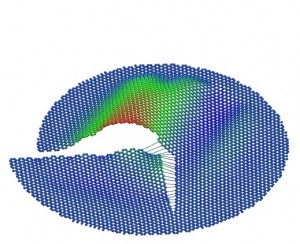
Molecular dynamics modeling of fracture of graphene
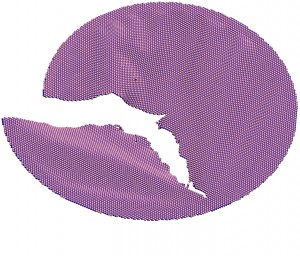
Molecular dynamics modeling of fracture of monolayer hexagonal boron nitride.
Using density functional theory (DFT), molecular dynamics and finite element method we investigate mechanical properties and failure characteristics of mono and few-layer two-dimensional materials. The fantastic physical, electrical and mechanical properties of two-dimensional materials have made them important for a variety of applications in a wide spectrum of fields such as supercapacitors, optoelectronics, spintronics, lithium ion batteries and nanocomposites. Understanding the mechanical properties of two-dimensional materials is crucial in their reliable usage in such sensitive devices. We envision that research on the mechanical properties of two-dimensional materials can complement the research on their electrical and physical properties and pave the way toward realizing industrial applications for such materials. Having this vision, we investigate the mechanical and failure properties of two-dimensional materials. We also will study the effect of defects such as grain boundaries and vacancies on the mechanical properties of these materials.